What is epigenetics?
There’s a lot we still don’t understand about how our genes work, and even well-meaning people can get in a mess over epigenetics
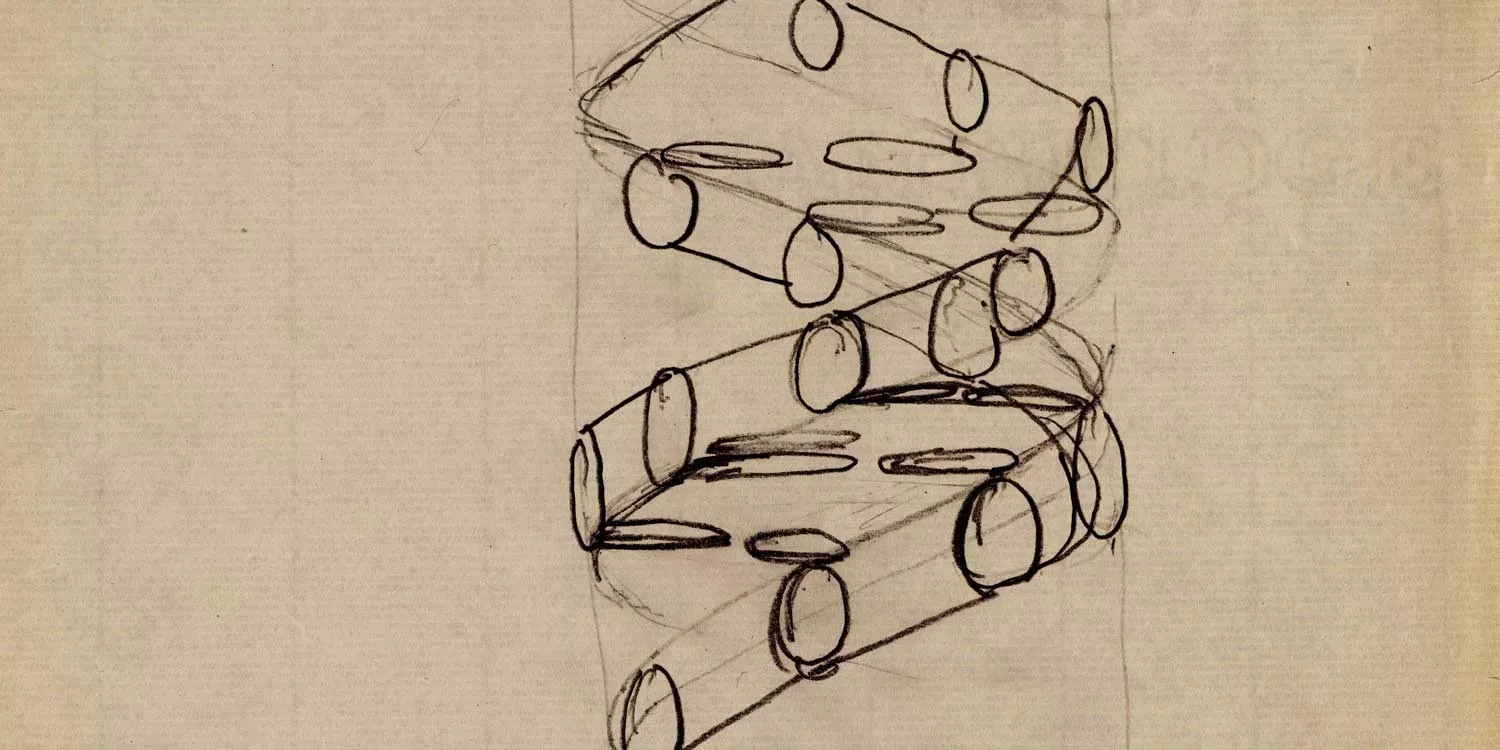
This article originally appeared in issue 2 of the Little Atoms magazine. To find similar articles and buy a copy, please click here.
In its simplest sense, epigenetics is the science of how genes get turned on and off. All the cells in your body (more or less) have the same set of 20,000-or-so genes. This is your genome – a set of biological recipes that your cells use to make the molecules that keep them functioning and keep you alive. But all your cells are not the same, so all the genes can’t be switched on all the time. Otherwise you’d look like some hideous blob of Silly Putty, and that would be awful. You have skin cells, liver cells, bone cells, brain cells, toenail cells – each type doing its own special job.
Clearly, different types of cells need to use particular suites of genes. We need the molecular recipes making sturdy keratin proteins to be used in skin cells, keeping the outside out and your insides in. Brain cells use genes encoding a crackling array of neurotransmitters and receptors, enabling you to read and (I hope) understand this article. But they certainly don’t need liver enzyme genes, so those get permanently switched off.
Different types of cells need to use particular suites of genes
What’s more, these on-and-off settings aren’t fixed. Genes can get activated or shut down during development as a foetus grows in the womb, depending on when and where they’re needed. Throughout our lives all sorts of genes are turned up or down in response to information coming in from the environment. Eaten a big dinner? You need to switch on the genes that make enzymes to break down the food and shuttle it into your body. Overdone it in the sunshine? Quick! Activate the damage-response genes to protect you from the damaging effects of the sun. (That’s what peeling sunburn is, by the way: skin cells that have activated a programme of suicide genes so they die rather than persisting, crippled and potentially cancerous, in the body.)
This is fundamentally how the interaction of nature (genes) and nurture (the environment) works. It’s nothing particularly weird, and it’s something that scientists have known about for decades. However, the word epigenetics has come to mean many things to many people, and for the pedants the biggest issue is one of semantics. In its purest sense – the way that the coiner of the term, Conrad Waddington, described back in the 1940s – epigenetics is “the branch of biology which studies the causal interactions between genes and their products which bring the phenotype into being”. In other words, how your genes and the molecules they make work together to make you, you.
What follows from this idea is the assumption that epigenetic information is somehow written into our genes by the environment and influences the way they work. And then there is the tantalising idea that these changes might be passed down the generations, from parent to child or even further. That’s the stuff that has got the science journalists and quantum peddlers most excited, but it’s also the least supported by evidence. Frustratingly, “it must be epigenetic” has become a catch-all for “we don’t really understand this”. So what do we know?
Genes are short stretches of DNA – the twisted ladder of life, often used by lazy advertisers as shorthand for “Oooh science!” DNA itself is a molecule made up of four chemicals (bases) strung together millions of times in seemingly endless permutations. They’re known by their initials A, C, T and G – short for adenine, cytosine, thymine and guanine – and it’s the order of these letters in a gene that determines that particular molecular recipe. Each one of your cells contains a staggering 2.2 metres of DNA, comprising roughly 3bn A, C, T and G letters – the same as 200 phone books. Furthermore, DNA doesn’t writhe naked and free within our cells. It’s wrapped around ball-shaped proteins, histones, and then coiled, supercoiled, crushed and squished into a structure in the middle of the cell, the nucleus, smaller than the head of a pin.
Since the 1970s, scientists have discovered that the histone proteins packaging our DNA can be modified with tiny chemical tags, each of which is supposed to convey a particular meaning. Some are the biological equivalent of a “no entry” sign, telling the gene-reading machinery of the cell to leave these genes alone. Others declare the underlying DNA open for business, so the genes in that region can be switched on. Then there’s DNA methylation – tiny chemicals called methyl groups that are directly glued onto the letter C in DNA at particular locations.
Hundreds of research groups around the world have spent the past couple of decades mapping patterns of histone modifications and DNA methylation in ever more detail around thousands of genes, monitoring how they change in response to various stimuli coming from inside and outside cells. Many, many papers have been published, and they are mostly very tedious. Every field, from cancer research to psychology, is busy detailing these so-called epigenetic modifications at their favourite genes. It’s the biological equivalent of stamp-collecting.
DNA doesn’t writhe naked and free within our cells
There has been a disturbing fixation on mapping patterns of DNA methylation, on the assumption that finding these tell-tale tags on genes means that they are switched off. But is DNA methylation or histone modification a cause – the actual thing that switches a gene on or off – or is it just a consequence of other underlying processes at work? To draw an analogy, is it like the locked door of a shut-up shop, or just the “closed” sign swinging against the windowpane, a visible readout of a more fundamental process at work? Most research shows that genes are activated by protein molecules called transcription factors, which sit on short stretches of DNA near genes and act as “control switches” to turn them on. Histone modifications may be important for locking in patterns of gene activity once the transcription factors have got them going, but there’s still a lot we don’t really understand about how it all works.
The lack of a clear understanding of the link between epigenetic modifications and gene activity has allowed an almost cult-like field of misunderstanding to spring up. The idea that “fiddling with the epigenetics” can turn genes on and off has seeped into pop science, and led to pseudoscientists like the New Age guru Deepak Chopra telling us we can forge our genes with the fire of our wills or by eating enough broccoli.
It may sound like a subtle or trivial difference but it’s actually a scientific minefield, as the author Siddhartha Mukherjee, a Pulitzer prize-winner, recently discovered. To promote his new book, The Gene, Mukherjee wrote a piece for the New Yorker magazine claiming that epigenetics is everything when it comes to gene activity. He was surprised to find himself publicly ticked off by a range of high-ranking scientists – mainly legendarily grumpy men – for misrepresenting the state of the science. The Epigenetics Kerfuffle, as biologist Jerry Coyne calls it, has even led to Mukherjee revising sections of his book to “increase clarity” (rather than admitting anything is actually wrong with it, of course).
This might sound like the kind of argumentative but inconsequential dick-waving that goes on in every sphere of academic and intellectual endeavour, but it matters when epigenetics is invoked to explain every single biological phenomenon we don’t yet understand. One of the biggest mysteries is something known as “transgenerational epigenetic inheritance”. It’s the concept underpinning all those headlines you read asking “Was Darwin wrong?”, the idea that epigenetic stuff (rather than the underlying DNA code itself) can transmit information, traits or even diseases from one generation to the next.
The big question is how does epigenetics work
Transgenerational epigenetic inheritance is often held up as scientific heresy, akin to a modern version of the French naturalist Jean-Baptiste Lamarck’s idea 200 years ago that physical characteristics accumulated during life can be passed on from parent to child. Think of the burly blacksmith, building up muscles over a lifetime and passing on both his trade and frame to his sons. This was easily disproven by the legions of mice whose tails were chopped off by Lamarckian believers, only to produce offspring with full-length tails. But there is good evidence from experiments with fruit flies, nematode worms and plants suggesting that this can happen to some extent, although not in the dramatic way that Lamarck thought.
Things get a lot more sketchy, however, when it comes to humans and other mammals. There are a couple of famous examples of transgenerational inheritance, very few of which have been pinned down to a solid epigenetic explanation. The most famous – the Dutch Hunger Winter – dates back to September 1944, in Nazi-occupied Holland. Despite the offensive that followed the D-Day landings, the Allied powers failed to free most of the Netherlands in late 1944, and the Germans imposed a savage blockade on their remaining occupied territory.
The resulting food shortages were desperate. At the peak of the famine many people were surviving on two slices of bread and half a sugar beet a day – a ration of around 400 calories. Some describe topping up this meagre diet with sliced tulip bulbs fried in engine oil or occasional squirts of artificial whipped cream. In May 1945, the Netherlands was liberated – the famine was severe but mercifully short. But because life and love continue in the hardest circumstances, some women in the famine-struck areas fell pregnant during the crisis, or were in the earliest stages of gestation. Others were further along, and some gave birth before the German blockade was lifted.
Every field of science is susceptible to fads
By poring through the surprisingly detailed medical records of the time, researchers have been able to find these babies and follow them through life. Initially, everything seemed to be OK, although mothers who were starved for the final few months of their pregnancy tended to have smaller babies. Children conceived during the later months of the famine but whose mothers enjoyed a better diet for the end of their pregnancy were born a normal weight. Later on in life, though, it started to become clear that all was not entirely well with these kids. They ended up more obese, with greater incidence of heart disease. They also aged more quickly, were more likely to die of cardiovascular problems, and perhaps had increased odds of developing schizophrenia. Finally, there were tantalising hints that some of these problems may pass further down the generations, affecting the grandchildren of the original starved mothers.
The big question now is how does it work? Researchers have found differences in DNA methylation at important genes involved in foetal growth in the Dutch children who were starved in the womb. But this doesn’t need to invoke any spooky transgenerational stuff. A foetus is still part of its mother – not a separate entity in a sealed box – and will switch genes on and off in response to signals coming from the outside world. And because the germ cells that will become the eggs and sperm that create the next generation are laid down very early on in development, this also explains the impacts on the grandchildren of the Dutch mothers.
It’s a phenomenon known as developmental programming. In the case of the Hunger Winter, the babies conceived during this time are sensing the lack of food in the environment and making changes in how they use the limited chemical building blocks provided by the mother’s starvation diet. They’re also modifying their gene activity to prepare them for a life of scarcity. Some people like to go further than the relatively simple idea of developmental programming, suggesting that extra information might be passing from one generation to the next through egg and sperm. However, it’s broadly thought that epigenetic marks – DNA methylation or histone modifications – get removed from germ cells, wiping the slate clean for the next generation. So it’s unlikely that this is the mechanism at work. Nevertheless, there have been a few intriguing papers over the past couple of years suggesting that tiny fragments of RNA (a chemical similar to DNA), hitching a ride in egg and sperm, might be transferring extra information from parent to child or even further. It’s still a slightly fringe idea, but one to watch.
All fields of science are susceptible to fads, and people have been quick to jump on epigenetics as an explanation for all the mysteries of life that we haven’t yet fully unraveled. Yet while it’s important to get to the bottom of the mechanistic nitty-gritty about the exact genes and molecules involved, there’s another angle that shouldn’t be ignored.
Although the impact of the Dutch Hunger Winter is measurable today, the effects aren’t drastic. This is because the Netherlands is a wealthy country with plentiful food and good public healthcare, so the affected children can (to a certain extent) catch up. But similar studies in other countries, notably in the developing world, tell a less positive story. Taken together, the evidence suggests that good nutrition and healthcare for women and girls – especially in early pregnancy or for women trying to conceive – are vital to ensure the long-term health of everyone in society. It’s not more epigenetics that we need, but more equality.